Viewpoint by Evgeniia Kirillova
The views expressed in this article are solely those of the author and she is not speaking on behalf of her employer.
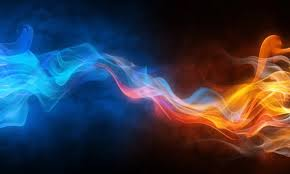
Abstract: The transition to a sustainable energy future requires a thorough understanding of the intricate dynamics within electricity, hydrogen and natural gas energy markets. Integrated models have emerged as essential tools to navigate this complexity, providing comprehensive insights into future energy landscapes and enabling prudent decision making. However, the integration of these energy systems presents unique challenges, requiring strategic capacity planning and co-optimisation to ensure seamless interactions. This paper explores the integration of the electricity, hydrogen and natural gas energy worlds within a co-optimisation framework, addressing practical issues and benefits for strategic planning. The co-optimisation model generates dynamic commodity pricing by linking, for example, the production of hydrogen to the hourly generation of electricity used to produce the same hydrogen through electrolysis. The utilisation of the produced hydrogen is in turn linked to the generation of electricity through combined cycle gas turbines (CCGT). Critical considerations include maintaining consistent units and currency, ensuring technology and price consistency, and addressing cumulative differences. The benefits of co-optimisation models extend to both companies and policy makers, facilitating better decision making by optimising capacity expansions, minimising costs and balancing economic efficiency with environmental impact. These models enable the seamless deployment of clean energy solutions, mitigate the risks associated with energy price volatility, and promote operational resilience. In summary, the integration of co-optimisation models provides a holistic approach to energy decision making, maximising the potential of electricity and hydrogen energy systems in the transition to a sustainable energy future.
Keywords: integrated modelling, energy transition, co-optimisation, interconnected systems, sustainable energy, market dynamics, fuel switching, capacity expansion, hydrogen integration, strategic decision-making
Преимущества использования решений в сфере моделирования кооптимизации для взаимосвязанных энергетических рынков
Аннотация: Переход к устойчивому энергетическому будущему требует глубокого понимания сложной динамики рынков электроэнергии, водорода и природного газа. Интегрированные модели стали важными инструментами для преодоления этой сложности, обеспечивая всестороннее понимание будущих энергетических ландшафтов, позволяя принимать разумные решения. Однако интеграция этих энергетических систем представляет собой специфические проблемы, требующие стратегического планирования производственных мощностей и согласованного подхода в моделировании для обеспечения точного взаимодействия всех элементов кооптимизиционной модели. В данной статье исследуется интеграция миров электроэнергии, водорода и природного газа в рамках кооптимизации, рассматриваются практические вопросы и преимущества для стратегического планирования. Модель кооптимизации формирует динамическое ценообразование на сырьевые товары, связывая, например, производство водорода с почасовой генерацией электроэнергии, используемой для производства этого же водорода путем электролиза. Использование произведённого водорода, в свою очередь, соединено с генерацией электричества посредством газовых турбин комбинированного цикла (CCGT). Важными факторами являются поддержание единообразия единиц измерения, технологий и валют, что обеспечит последовательность в контексте ценообразования и позволит устранить кумулятивные различия. Преимущества моделей кооптимизации актуальны как для компаний, так и для законодателей, так как данные модели способствуют более эффективному принятию решений за счёт оптимизации расширения мощностей, минимизации затрат и уравновешивания экономической эффективности и воздействия на окружающую среду. Эти модели позволяют беспрепятственно внедрять решения в области экологически чистой энергетики, снижать риски, связанные с волатильностью цен на энергию, и повышать эксплуатационную устойчивость. Таким образом, интеграция моделей кооптимизации представляет собой целостный подход к принятию решений в сфере энергетики, максимизируя потенциал электроэнергетических и водородных энергетических систем при переходе к устойчивому энергетическому будущему.
Ключевые слова: взаимосвязанные системы, динамика рынка, интеграция водорода, интегрированное моделирование, кооптимизация, переход на другой вид топлива, принятие стратегических решений, расширение мощностей, устойчивая энергетика, энергетический переход
A Holistic Approach to Navigating the Complexities of Energy Market Integration in Co-optimisation Modelling
Navigating the complex dynamics of energy markets is akin to deciphering a labyrinth of interconnected systems, where every decision has ripple effects across the landscape. Recent studies highlight the paramount importance of integrated models over isolated simulations in navigating the complexities of energy transitions[1], which have long since moved beyond planning or creating simulations of individual standalone energy markets.
Some authors note that the need to coordinate data from multiple energy market actors can make co-optimisation of non-isolated energy systems more complex. However, they also point out that practical applications of these co-optimisation approaches show significant improvements in the operational efficiency of the whole energy system and reductions in carbon emissions.[2]
More and more researchers[3] are highlighting that carefully designed co-optimisation models mainly aim to achieve several key objectives: minimising total costs, improving the integration of energy conversion and storage technologies, developing the knowledge of different market behaviours, and understanding market interconnectedness.
In addition, current European network organisations, such as the European Network of Transmission System Operators for Gas (ENTSOG), emphasise the importance of testing “Interlinked Models”. These models integrate hydrogen, electricity and other gas markets to understand the impact of the energy transition on the size and design of future energy networks.[4] The recently published Interlinked Model (ILM) 2024 progress report[5] highlights several benefits of developing co-optimisation models. The report notes that this direction of energy modelling is in line with the TEN-E Regulations: Regulation (EU) 2022/869 on guidelines for trans-European energy infrastructure, which requires coordinated system planning across sectors. Co-optimisation energy modelling represents a significant step forward in understanding and managing an increasingly integrated energy system, which is crucial for achieving the EU’s long-term energy and climate goals.
However, in the midst of this complexity, strategic capacity planning within an integrated framework takes centre stage. Models that convolutely connect together market interactions, including the dynamics of fuel switching in generation capacity, provide a comprehensive view of the future energy landscape and enable more prudent decision making.
Delving deeper into the realm of energy markets, the electricity sector operates within its own unique set of rules and dynamics. With a strong focus on hourly production and dispatch, the electricity market requires bespoke models that reflect day-ahead electricity markets, meticulously analysing bidding strategies and generator capacity across a range of energy sources, from renewables to traditional fossil fuels.
As the world moves towards a cleaner energy future, hydrogen is emerging as a key player in the transition. To understand the nuances of the hydrogen market, it is necessary to look at the various sources of supply. Among these, blue hydrogen, derived from Steam Methane Reforming (SMR) plants, embodies the stability of the natural gas world, characterised by relatively stable pricing with contracted volumes.
In contrast, green hydrogen, which is produced by power-to-x (P2X) electrolysers, represents a paradigm shift, relying entirely on intermittent renewable electricity generation. These different sources of supply must seamlessly match demand volumes at national hubs connected by hydrogen pipelines, with flexibility provided by additional storage capacity to accommodate fluctuations in demand and price adjustments during peak periods.
Although hydrogen, electricity and natural gas are integral parts of the future energy landscape, they are measured in fundamentally different units. Electricity, typically measured in kilowatt hours (kWh), quantifies the amount of energy consumed over a period of time, reflecting the flow of electrical charge through a circuit. Hydrogen, on the other hand, is often measured by volume, typically in cubic metres (m³), or by mass, such as kilograms (kg) or metric tonnes (t). This distinction arises from the different nature of their respective properties and applications. While electricity represents the flow of electrons through a conductor, hydrogen is a gas that can be stored and transported in various forms, such as compressed gas or liquid. Understanding and reconciling these differences in units of measurement is essential for effectively integrating hydrogen and electricity into energy models and systems, and for ensuring accurate analysis and decision-making in the transition to a sustainable energy future.
In addition, natural gas, an important player in the energy landscape, is measured in units such as cubic metres (m³) or cubic feet (ft³), reflecting its volume when stored or transported. Unlike hydrogen and electricity, natural gas is a fossil fuel composed primarily of methane and is widely used for power generation, heating and industrial processes. Its different properties and applications further emphasise the need to understand and accommodate different units of measurement within energy modelling frameworks.
In addition, liquefied natural gas (LNG) represents an additional dimension to the measurement and use of natural gas. LNG is natural gas that has been cooled to a liquid state for ease of storage and transportation and is typically measured in metric tonnes (t) or cubic metres (m³) in its liquefied form. This process allows natural gas to be transported over long distances to regions without pipeline infrastructure, facilitating global trade and increasing energy security. As LNG plays an increasingly important role in the global energy market, understanding its unique units of measurement and logistical considerations is critical to comprehensive energy planning and sustainable development initiatives. In the context of energy modelling and system integration, the consideration of LNG’s unique characteristics alongside hydrogen and electricity further underlines the complexity and importance of reconciling different units of measurement for effective decision-making in the transition to a more sustainable energy future.
As we navigate the transition to a sustainable energy future, harmonising the units of measurement of hydrogen, electricity and natural gas will be paramount for holistic energy planning and decision-making.
Creating a cohesive, integrated model that fully captures the nuances of different energy markets is a formidable task. Each market operates within its own ecosystem, governed by unique rules, dynamics and nuances. From the hourly nuances of electricity generation and dispatch, to the stability of contracted volumes in the natural gas world, to the renewable-dependent nature of hydrogen production, every detail matters.
Failure to account for these nuances risks compromising the effectiveness of the model and, consequently, the integrity of the decision-making process. The importance of attention to detail cannot be overstated. Only by carefully considering the specifics of each market can we ensure the seamless interplay necessary for a sustainable energy future. Embracing this complexity and seeking a holistic understanding paves the way for informed decision-making and the realisation of a greener, more resilient energy landscape.
Practical Issues Needed to Be Awarded and Solved prior of Designing the Model
The design of the co-optimisation model depends on the establishment of complex linkages. These links weave together the dynamic price of natural gas, driven by fundamental market movements, and the price of hydrogen, determined by two different production methods (SMR and electrolysis) alongside Combined Cycle Gas Turbines (CCGT). The objective is twofold: firstly, to manage the supply of electricity to electrolysers (P2X units) for green hydrogen production, while meeting baseload electricity demand. Secondly, it needs to be checked that CCGTs receive hydrogen from both SMR and P2X sources, each with its own market fundamentals. At the same time, hydrogen fuel, which is subject to dynamic pricing at the national hydrogen hubs, must be delivered efficiently to the hydrogen-fuelled CCGTs, and this requires accurate regional modelling for seamless connections. A similar process applies to natural gas generation units, with natural gas being connected to both natural gas power generators and SMR units.
Developing a co-optimisation solution for such a model presents its own set of challenges. It’s like conducting a symphony in which a classical orchestra meets modern electronic beats – balancing the levels of granularity, time scales and volatility risks inherent in two different energy domains. Bridging these divides isn’t just about finding common ground; it’s about embracing the inherent complexities and intricacies of both worlds.
To ensure the accuracy and reliability of our modelling approach, we need to address several critical considerations:
1. Unit and heating value consistency. Maintaining consistency in units across all parameters is critical for accurate comparisons and calculations. In particular, for prices, volumes and energy content, consistency in units and heating values, whether high heating value (HHV) or low heating value (LHV), is paramount when dealing with different fuels.
2. Currency and base year consistency. Using the same currency and base year throughout the modelling process facilitates meaningful comparisons and ensures consistency of price data and economic parameters across different model segments.
3. Price mechanisms and technological consistency. Ensuring consistency of pricing mechanisms across different parts of the model is critical to maintaining accuracy in forecasting and analysis. For example, if a disaggregated model uses a specific natural gas pricing mechanism for gas-fired plants, it is important to verify that the natural gas price nodes in the co-optimisation model are connected correctly and reflect what was intended. Similarly, maintaining consistency between technologies in the co-optimisation model, including the non-fuel portion of operating costs, is key to accurate assessments. For example, if hydrogen-methane reforming is linked to steam reforming rather than autothermal reforming in a disaggregated model, this distinction should be appropriately reflected in the linked model.
4. Awareness of cumulative differences. Recognising the potential impact of accumulated differences is critical, as even small discrepancies can lead to significant errors in modelling results. Proactively addressing these differences improves the accuracy and precision of our analyses and avoids potential pitfalls.
By following these guidelines and carefully addressing each aspect, we can strengthen the integrity of our modelling framework, paving the way for reliable insights and informed decisions in the dynamic energy landscape.
Benefits for Strategic Planning
The integration of co-optimisation models and solutions provides a flexible approach to the complexities of energy markets, benefiting both companies and policy makers. These models combine the dynamics of different natural energy markets, providing a comprehensive view of the energy landscape, which in turn facilitates better decision making. By prioritising strategic capacity planning within an integrated framework, they enable detailed analysis of market interactions and fuel switching dynamics that are critical for effective energy transitions.
These models have demonstrated superior performance compared to stand-alone models, resulting in smoother price curves and more optimal capacity expansions. They excel at simultaneously optimising different facets of the energy landscape, including generation, transmission and storage. By harmonising cost targets across all components, market participants can determine the optimal mix of energy sources to meet demand while minimising costs. Essentially, they foster a dialogue between different fuel types, enabling a thorough assessment of how different renewable technologies can be seamlessly integrated.
Taking into account factors such as intermittency, geography and grid constraints, these models enable policymakers to develop strategies that facilitate the seamless deployment of clean energy through diverse solutions. Navigating energy markets is similar to navigating complex ecosystems with myriad stakeholders, ranging from producers and consumers to grid operators. As a result, energy price volatility and supply disruptions pose risks to businesses and utilities. These models enable companies to assess risk scenarios and develop strategies to mitigate them, such as optimising investments in energy storage to reduce exposure to price fluctuations.
They also help to determine the optimal timing of such actions, thereby saving costs and strengthening grid stability. In essence, these energy models provide policy makers and market participants with data-driven insights that enable them to make informed decisions that balance economic efficiency, environmental impact and operational resilience, all based on a single solution to the equation.
Conclusions
The inclusion of hydrogen in the energy market has led to smoother price fluctuations with fewer sudden spikes, fostering a more stable and balanced energy ecosystem. This success is due in part to the inclusion of a fully modelled hydrogen system that includes both blue and green hydrogen production methods. In addition, the use of natural gas for SMR processes has played a significant role in diversifying hydrogen production sources and reducing electricity consumption. In particular, the integration of blue hydrogen production with carbon capture and storage (CCS) technologies highlights its role in mitigating carbon emissions and promoting sustainable energy practices.
Through co-optimisation modelling solutions, we can strategically fuse all the pieces of the energy puzzle to maximise their potential within the landscape. By simultaneously addressing multiple objectives such as cost minimisation, emissions reduction and resource optimisation, these models provide a holistic approach to energy decision making.
The dynamics of energy markets present a complex but manageable challenge if the model is well designed, with the integration of different energy worlds emerging as a pillar for strategic planning in the transition to a sustainable energy future. By creating carefully designed links between the different energy worlds, co-optimisation models provide a comprehensive understanding of the interactions between markets and the dynamics of fuel switching and energy transition process.
About Evgeniia Kirillova
Evgeniia Kirillova is an energy strategy specialist with over 8 years of experience in senior positions at prominent energy companies. Currently a Senior Energy Market Analyst at a software company developing an energy modelling and analysis platform, Evgeniia is involved in the development of forecasting and optimisation models tailored to global energy markets, with a particular focus on natural gas, hydrogen and electricity. At Gazprom, Evgeniia led strategic projects, including the development of a mathematical application that improved the accuracy of long-term calculations for natural gas flows. Evgeniia holds a Master’s degree in Corporate Finance and Risk Management from Université Paris Dauphine – PSL.
Address for correspondence:
evgekia@gmail.com
[1] IRENA (2022), World Energy Transitions Outlook 2022: 1.5°C Pathway, International Renewable, Energy Agency, Abu Dhabi, https://www.irena.org/Digital-Report/World-Energy-Transitions-Outlook-2022.
[2] Géremi Gilson Dranka, Paula Ferreira, A. Ismael F. Vaz, “A Review of Co-optimization Approaches for Operational and Planning Problems in the Energy Sector,” Applied Energy, Volume 304, 2021, 117703, ISSN 0306-2619, https://doi.org/10.1016/j.apenergy.2021.117703.
[3] Haoxin Dong, Zijing Shan, Jianli Zhou, Chuanbo Xu, Wenjun Chen, “Refined Modeling and Co-optimization of Electric-hydrogen-thermal-gas Integrated Energy System with Hybrid Energy Storage,” Applied Energy, Volume 351, 2023, 121834, ISSN 0306-2619, https://doi.org/10.1016/j.apenergy.2023.121834.
[4] ENTSOG and ENTSO-E Publish Their Joint Electricity and Hydrogen Interlinked Model 2024 Progress Report for Public Consultation, 7 May 2024, https://www.entsoe.eu/news/2024/05/07/entsog-and-entso-e-publish-their-joint-electricity-and-hydrogen-interlinked-model-2024-progress-report-for-public-consultation/.
[5] Progress Report on Interlinked Modelling. The Cross-sectorial Integration of Energy System Planning, ENTSOG and ENTSO-E. https://www.entsog.eu/sites/default/files/2024-05/entsos_ILM_progress_report_240430.pdf.